All published articles of this journal are available on ScienceDirect.
Relationship between Cortical Excitability and Complex Reaction Time
Abstract
Introduction:
The purpose of this investigation was to investigate the relationships between cortical excitability and complex reaction times (RT).To carry out this study, we performed transcranial magnetic stimulation (TMS) to test cortical excitability and the Posner paradigm to investigate the RT and errors. Investigation of motor cortex excitability and reaction time.
Methods:
Twenty male right-handed participants were chosen for this investigation (Age: 23.5±2.1 years; Height 177.1±2.8 cm; Body mass 73.2±3.3 Kg).
Results:
A significant positive correlation emerged between resting motor threshold (rMT) and RT and between motor evoked potential (MEP) latency and RT(p<0.001). The results also show a significant positive correlation (p<0.001) between rMT and the percentage of errors and a significant positive correlation (p<0.05) between MEP latency (ms) and the percentage of errors. The main results of the study showed that subjects who showed lower motor activation thresholds were able to respond faster and they also showed a significantly lower error rate compared to subjects who showed higher motor activation thresholds.
Conclusion:
To the best of our knowledge, our study seems to confirm the presence of a relationship between neuro-physiological parameters (MEP latency and rMT), RT and percentage of correct answers.
1. INTRODUCTION
Our knowledge of the processes underpinning learning and the accomplishment of motor tasks has significantly advanced over the past 10 years. A notable advancement has been the development of non-invasive electrophysiological procedures like transcranial magnetic stimulation (TMS), which uses powerful magnets placed near the skin to electromagnetically stimulate brain tissue electromagnetically [1]. This method induces a relatively tiny and brief change in the electrical activity, which can be used to stimulate and investigate the operation of the brain's circuits and neural connections. Numerous research have been conducted in recent years to examine the connection between brain activity and muscular reaction. In this regard, research on athletes was crucial for understanding the mechanics behind the performance of motor activities. The corticospinal excitability of karate practitioners was found to be greater than that of sedentary people, showing that exercise alters the interactions and balance between inhibitory and facilitatory circuits, which define the primary motor cortex's (M1) final output. Furthermore, sports-specific neurophysiological configurations may be supported by the strong association between corticospinal excitability and coordination performance [2, 3]. In a different study, the authors discovered that karate practitioners had faster reaction times (RT), lower resting motor thresholds (rMT), shorter motor evoked potential (MEP) latency, and higher MEP amplitude. In addition, they discovered that there was a significant positive linear correlation between RT and rMT, between RT and MEP latency, and between RT and MEP amplitude [4, 5]. The differences between the trained and untrained groups in this study's findings imply that corticomotor projections can become functionally malleable with long-term reinforcement and helpful motor practice [6, 7]. The differences discovered in earlier research may have resulted from training-induced improvements in cortical excitability. In fact, the adult brain can change how it is organized through physiological processes like practicing easy actions repeatedly. However, in a dynamic environment, brain plasticity allows the nervous system to ensure that the muscles are correctly activated to achieve the behavioral objective, and, more recently, a genetic component for brain plasticity has been found [8, 9]. Numerous studies have shown that structured physical training is an excellent strategy to improve motor skills and induce changes at the cortical level [10-12]. In this regard, it has been observed that in physical activity programs aimed at improving coordination skills by observing target movements, an increase in cortical excitability is observed, which could lead to an improvement in learning processes [13, 14]. A recent investigation has shown that simple RT is related to the level of cortical excitability and that increasing the complexity of a movement consequently increases the RT. These results highlight that cortical excitability influences both RT and decision-making processes [15, 16].
Being able to pay attention is a crucial life ability that is directly linked to memory, learning, perception, and executive function. The fundamental job of attention is to choose and select a portion of the available stimuli while simultaneously suppressing irrelevant information. Focused attention is the ability to respond clearly to tactile or visual information. Selective attention is the ability to retain a behavioral or cognitive set in the face of competing or distracting stimuli. In contrast, sustained attention is the capacity to maintain a steady behavioral response throughout continuous and repetitive activities. [1-3]. However, corticospinal differences in excitability may also be problems of previous studies in a neuron-enhanced exciting state of complex neuron tasks. It would be fascinating to find out whether cortical excitability may influence RT in light of the rise in RT associated with stimulus difficulty. Thus, the purpose of this research was to investigate the presence of relationships between cortical excitability, assessed using TMS, and complex RT, assessed using the Posner test. The cortical excitability parameters investigated are rMT (%) and MEP latency, while, the Posner test were performed in order to investigate the RT and the percentages of correct answer [15, 17].
2. MATERIALS AND METHODS
2.1. Participants
The study was authorized by the local institutional ethics committee (Azienda Ospedaliera-Universitaria “Ospedali Riuniti,” Foggia, Independent Ethics Committee; protocol number that was attributed by the ethics committee: 116/CE/2011, 14/11/2011). All participants in the trial were informed verbally and in writing of any potential hazards and discomforts, and it was made clear to them that they might leave the study at any moment. Additionally, a medical examination verified that there were no risk factors or other contraindications in accordance with the safety and advice for using TMS, as well as the absence of psychoactive or vasoactive pharmaceutical assumptions [18]. All subjects gave their written consent before participation. No one was disqualified from the process since none of the individuals who were enrolled for the trial had any contraindications. The volunteers chosen for the experiment were required to refrain from exercise and consume less caffeine for 24 hours before the trial began. Twenty right-handed [19] males were recruited in this study (age: 23.5±2.1 years; height 177.1±2.8 cm; body mass 73.2±3.3 Kg). There were no differences between anthropometric parameters in subjects involved in this study.
All subjects were sedentary, which was defined as engaging in ≤60 min of exercise/week [20]. All subjects performed a Posner test and subsequently underwent a TMS procedure.
2.2. Study Design
The subjects recruited for the study were invited to the Physiology Laboratory of the University of Foggia. Upon entering the laboratory, each subject was explained the entire experimental procedure, which began after the signing of the informed consent. Before the beginning of the session, the subjects were explained how to carry out the Posner test. To ensure that all study subjects understood how to perform this test, a non-study subject showed them how to perform it. The subjects did not perform Posner tests to avoid a training effect that could have distorted the results. Subsequently, the tests were performed with this temporal sequence: detection of anthropometric parameters, positioning on the chair, identification of the RMT and recording of twenty stimuli for MEP analysis, Posner tests (Fig. 1).
2.3. TMS and Electromyographic Recording
The recording session was conducted between 9.30 and 11.30 in order to reduce any potential circadian influence. A DuoMag XT-100 Transcranial Magnetic Stimulator was placed over the left motor cortex of the participant while they were seated in a quiet room, and a 70-mm figure-of-eight coil was used to measure the excitability of the motor cortex. Right first dorsal interosseus (FDI) muscle surface recording electrodes were positioned over the muscle. The coil's handle was kept tangential to the scalp and tilted backward at 45 degrees from the midline by a mechanical arm while the stimulus was being delivered [21, 22]. The SofTaxic navigation system was used to locate the stimulation on each subject's scalp (E.M.S. Italy, http:// www.emsmedical.net). The resting motor threshold (rMT) was defined as the lowest stimulator intensity that produced a motor-evoked potential with a peak-to-peak value greater than 50 V in at least five out of ten consecutive trials. The literature [23] states that the stimulation can be increased or decreased in stages of 1-5% of the maximum output intensity to reach the desired threshold intensity. Individual rMT for left motor cortex stimulation was established using a set technique [23, 24]. After the identification of the rMT, 20 stimuli were collected for each subject and averaged. A ground electrode was placed on the forearm's dorsal area using the standard belly-tendon montage, and surface electrodes (1 cm in diameter) were placed over the corresponding joint or tendon and in line with the FDI muscle (active electrode) and muscle itself (reference electrode). The EMG signals were examined using a high pass filter with a cutoff frequency of 0.1 Hz (Acknowledge software, version 4.1, BIOPAC Systems, Inc., CA, USA). The magnetic stimulator was connected to the PC and interfaces with the EMG recording software. The stimulator sends a square wave signal (Trigger) each time it is activated. So, on the EMG trace, first the trigger was shown and immediately after the muscle response. The latency time was considered the time between the trigger itself (onset of the square wave) and the start of muscle response. Raw EMG signals were processed and analyzed (Acknowledge software, version 4.1, BIOPAC Systems, Inc., CA, USA) with a high pass filter (cutoff frequency: 10 Hz). For the RMT condition, twenty responses were averaged. We tested the protocol on the left cortex (M1) for all subjects.
2.4. Posner Test
The participants were seated in chairs and instructed to place their hands on a laptop's keyboard. They maintained their focus on a tiny white cross stimulus that filled 0.7° of the visual angle and was displayed in the center of a computer screen that was situated 80 cm away. A cue stimulus that was presented for 200 milliseconds (ms) at the start of the trial randomly (50%) indicated either a left or right side position along the horizontal meridian. The cue stimuli was a little white rectangle that either overlapped the left or right horizontal segment of the fixation cross and spanned roughly 0.2° of the viewing angle. After a 2-sec stimulus start asynchrony, a target letter, either L or T (each with 50% probability), was presented for 70 ms in either the left or right location, 0.7 degrees of visual angle from the fixation point. In 50% of the trials, the letters were shown in their canonical upright position; in the other 50%, they were rotated 180 degrees around the vertical axis. Each letter had a 0.7° viewing angle diameter. In 80% of the trials, the target stimulus appeared at the cue's stated location (valid trials), whereas in 20% of the trials, it appeared at the cue's opposite location (invalid trials) [15]. A mask stimulus (130 ms duration) comprised of all the potential line segments in the letter stimuli L or T was flashed immediately after the target stimulus to halt sensory processing. The subjects were taught to pay close attention as different stimuli were displayed on the computer screen. Additionally, when the letter T appears on the screen, press the left keyboard button (the Letter A key), and when the letter L appears on the PC screen, press the right keyboard button (the Letter L key).
It was balanced among subjects to assign the 'target' stimulus (T or L) to the particular key for reaction (A or L). This approach ensured that the center cue only provided information on the stimulus' location and not any instructions for how to respond. Making sure that the preparatory steps were visuospatial and unrelated to motor function is crucial [22]. For behavioral analysis, the response's RT and accuracy were collected.
2.5. Statistical Analysis
Statistical analyses were performed by GraphPad 6 Software, Inc., for Windows, version 6.01. The data are presented as mean (M) ± standard deviation (SD), and statistical significance was set at p < 0.05. The Shapiro–Wilk test was used to check the normal distribution of variables. Linear regression analysis was performed to investigate the relationship between the cortical excitability parameters and the results of the Posner test.
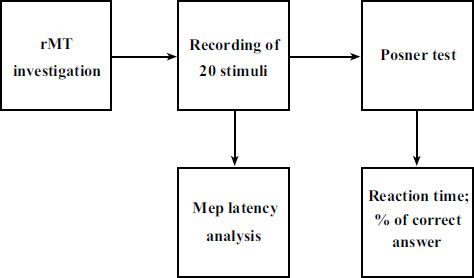
3. RESULTS
No discomfort or adverse effects during the TMS procedure were noticed or reported (Table 1).
Table 1.
- | errors% | RT (ms) | MEP Latency (ms) | rMT% |
mean | 9.35 | 419.29 | 25.5 | 51.45 |
sd (±) | 3.63 | 89.12 | 1.32 | 5.33 |
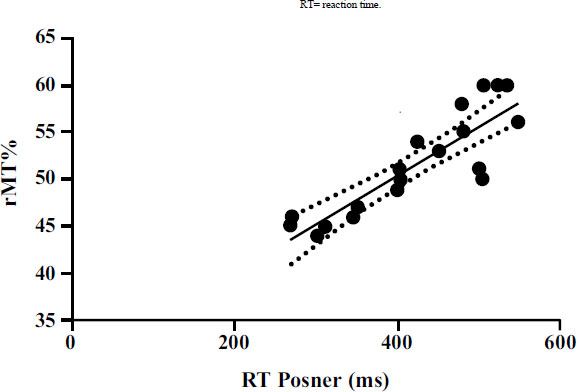
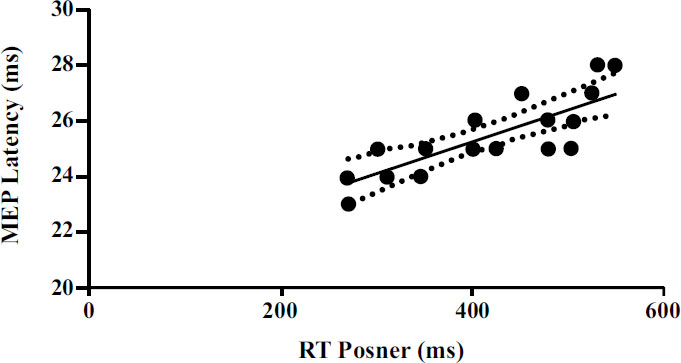
Furthermore, significant correlations emerged between the neurophysiological parameters detected by TMS and the parameters detected by the Posner test. A significant positive correlation (p<0.001) emerged between rMT and RT (Fig. 2). Moreover, a significant positive correlation (p<0.001) emerged between MEP latency and RT (Fig. 3).
We also performed a linear regression to investigate the possible relationship between cortical excitability and MEP latency with the percentage of errors committed by the subjects during the execution of the Posner test. The results show a significant positive correlation (p<0.001) between rMT and the percentage of errors (Fig. 4). The results also show significant positive correlation (p<0.05) between MEP latency (ms) and the percentage of errors (Fig. 5). The results of linear regression are shown in Tables 1 and 2.
Parameters | R2 | F | 95% C.I. | Best-fitValues | P |
---|---|---|---|---|---|
rMT% vs RT (ms) | 0.76 | 56.68 | 0.037 to 0.066 | 0.052 ± 0.006 | <0.001 |
MEP latency vs RT (ms) | 0.59 | 25.32 | 0.006 to 0.016 | 0.011 ± 0.002 | <0.001 |
rMT% vs errors% | 0.61 | 28.58 | 0.697 to 1.600 | 1.149 ± 0.214 | <0.001 |
MEP latency vs errors% | 0.26 | 6.37 | 0.031 to 0.340 | 0.185 ± 0.073 | <0.05 |
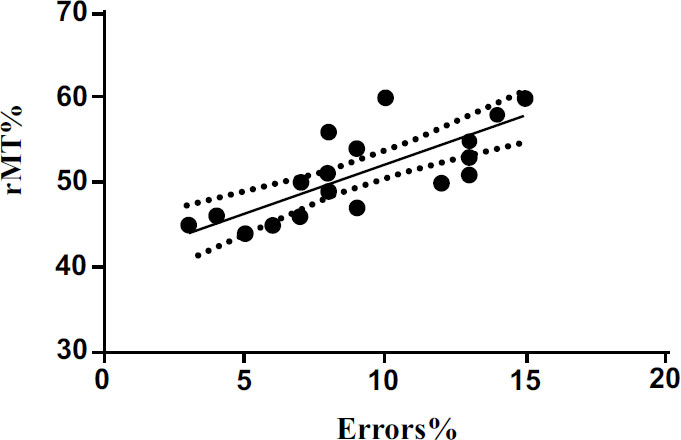
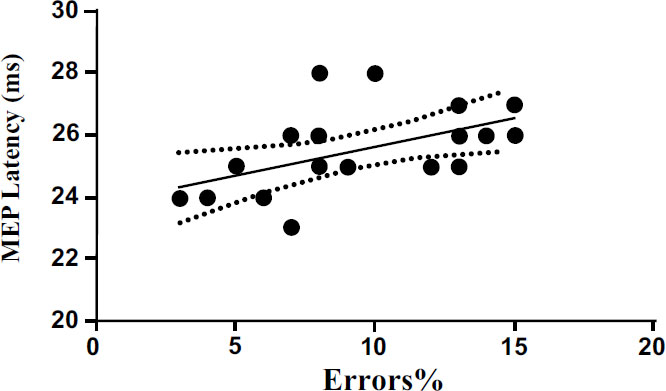
4. DISCUSSION
In this study, we tested the possible relationships between cortical excitability, with the related electrophysiological parameters, and the times of complex RT detected by a neuropsychological test often used to evaluate attention [25-27]. The main results of the study showed that subjects who showed lower rMT were able to respond faster and they also showed a significantly lower error rate compared to subjects who showed higher motor activation thresholds. We also had similar results with regard to the neurophysiological parameters related to TMS [28]. In fact, the subjects who showed shorter MEP latency were able to respond faster and also showed a percentage of errors significantly lower than the subjects who showed longer MEP latency [29]. In daily life, we constantly witness the control of actions and motor responses to ensure that they are suitable for multiple contests [23, 24], and these situations reflect the so-called concept of the “perception-action cycle”, introduced by Fuster (2003), which highlights the continuous interaction of perceptual neural networks with the executive component, presented by the motor cortex, which interacting with each other are able to perform the movements correctly [30]. As previously described, one of the most important consequences of these relationships is the ability to make a response that is appropriate to the perceived stimulus [26]. The results of our study seem to be in differential agreement with other studies in which the importance of neural networks has emerged. Which are able to analyze information correctly, and establish mature predictions on the sensory inputs of the neural network in order to be able to make quick and precise answers [31, 32]. After the meetings with real stimuli, the neural networks modify the synaptic weights in order to make better predictions of the environment in the future [27]. As widely shown in the literature, quantifying the RT and the percentage of correct responses of a motor task allows us to obtain information about the specific cognitive functions directly involved [28, 29]. Moreover, as showed in a previous study, lower RT is correlated to greater neural activations in young subjects who, therefore study, are able to obtain high performances in complex motor tasks. An important recent review has highlighted that the use of brain stimulation protocols during the execution of different motor tasks is able to reduce RT [1].
According to a previously published study, the frontal lobe regulates cognitive performance and the M1 cortex and frontal lobe have a physically hierarchical connection. The M1 and cognitive regions of the monkeys' frontal lobes showed single-cell activity both before and during the execution of coordinated movement, according to other studies [33-37]. They demonstrated complex physiological connections and linkages between the frontal lobe and M1 cortex [34-38]. According to Friston (2002), precise anatomical and functional connectivity underpins the context-dependent interactions across many brain areas that support sensory, motor, or cognitive functions [35-39]. This supports the theory that the frontal lobe, which regulates cognitive function, and the motor cortex are physically and functionally connected. According to this study, there seems to be a relationship between cortical excitability and RT, as shown in our investigation. Furthermore, positive effects on cognition related to RT were evident after applying brain stimulation protocols and in particular, a significant reduction in RT during learning and memory and executive functions / tasks was observed [30]. However, the situation is different when the response is not in series but carried out in parallel with the processing of the stimulus [33-36]. In other words, there are differences between simple RT and the RT that provide choices. In this regard, Ortiz et al., have shown that in subjects who perform the movement of one of the two hands linked to the type of stimuli, the potentials of premovement occurred first contralateral to the hand that had to move more frequently, even before the movements of the other hand. In this study, the authors could suggest that response selection and motor pre-activation occur in parallel [37, 38].
CONCLUSION
In conclusion, to the extent that we are aware, our study seems to show a relationship between neurophysiological parameters, RT and percentage of correct answers, unlike what has already been demonstrated in a previous study [39, 40]. Indeed, in this case, we have observed that improved cortical excitability also influences decision-making processes. Therefore, in the future, other studies could be conducted to evaluate the possibility of introducing different subjects to non-invasive brain stimulation protocols, such as TMS, to increase cortical excitability in order to obtain an improvement in performance. Furthermore, it would be advisable to increase the number of subjects and evaluate the same parameters in different populations to confirm the results obtained. For example, studies could be carried out to verify possible relationships, linked ages, between the parameters analyzed in this study. Another aspect to take into consideration is that in our study, we analyzed only two parameters (rMT and MEP latency) that can provide us with indications about cortical excitability. It would be advisable to carry out further studies to confirm the integrity of our data, analyzing other parameters and using other instruments (EEG and functional magnetic resonance) in order to provide us with ever more precise data regarding the relationships between excitability and complex reaction times. In fact, since cortical excitability is defined as the intensity of a cortical neuron's response to a given stimulation, reflects neuronal reactivity and response specificity, and is thus a fundamental aspect of human brain function [32], expanding the field of study can undoubtedly result in interesting data that can improve public health and give us in-depth knowledge about brain function.
LIST OF ABBREVIATIONS
(RT) | = Reaction Times |
(TMS) | = Transcranial Magnetic Stimulation |
(MEP) | = Motor Evoked Potential |
(M1) | = Motor cortex's |
ETHICS APPROVAL AND CONSENT TO PARTICIPATE
The study was authorized by the local institutional ethics committee (Azienda Ospedaliera-Universitaria “Ospedali Riuniti,” Foggia, Independent Ethics Committee; protocol number that was attributed by the ethics committee: 116/CE/2011, 14/11/2011).
HUMAN AND ANIMAL RIGHTS
No animals were used that are the basis of this study. All procedures performed in studies involving human participants were in accordance with the ethical standards of institutional and/or research committees and with the 1975 Declaration of Helsinki, as revised in 2013.
CONSENT FOR PUBLICATION
Informed consent was obtained from all participants of this study.
AVAILABILITY OF DATA AND MATERIALS
The data and supportive information are available within the article.
FUNDING
None.
CONFLICT OF INTEREST
Dr. Giovanni Messina is the Editor in Chief and Dr. Rita Polito is the Associate Editorial Board member of the journal The Open Neurology Journal.
ACKNOWLEDGEMENTS
Declared none.